- Fibromyalgia and Eye Pain - 29 January 2025
- Hyperalgesia and Fibromylagia - 24 January 2025
- Can You Be Hypermobile but Not Flexible? - 26 December 2024
If you would prefer to listen to this article, please click below.
If you’re reading this, then chances are you’ve been on quite the journey with your hypermobile body over the last few years. You’ve probably heard it all before, the endless advice about building muscle to stabilise those wobbly joints. However, let’s be real, it’s not always that straightforward, is it?
A lot of the time some of the advice just doesn’t make sense, or you have already tried it and it just didn’t really help.
How are you supposed to take the advice of building muscle to support, let’s say, a shoulder joint, when simply holding a cup subluxate/dislocates the joint?
Likewise, there are a lot of frustrations and unique challenges that come with hypermobility and connective tissue disorders, and exercising with hypermobility, well, that’s a whole other kettle of fish.
It’s high time we talk about exercising in a way that’s not just about pumping iron and strength focused because honestly, that’s really only the very tip of the iceberg. Today, I want to give you a new lens to look through, so that you can view exercising for hypermobility the same way I see it…..the magnificent, complex, multifactorial beast, that she is.
This article covers:
ToggleWhy should you be exercising if you have hypermobility or Ehlers-Danlos syndrome?
Exercise for those with hypermobility isn’t just a routine; it’s a lifeline. It’s about retraining our bodies to understand and adapt to the unique challenges the world throws at us, and helping to manage symptoms the best we can.
As you already know, having hypermobility can make our joints pretty unpredictable, leading to instability, discomfort, and pain, from relatively simple things. Exercise that’s tailored to our specific needs and goals, helps in building us a foundation of health that goes much beyond just muscle strength, but pushes into mental and social health side of things that the conditions bleeds through to effect.
What I mean by this, is that in recent years, a strong correlation between hypermobility and anxiety has been established. And, whilst the exact mechanism remain unknown (for now, Dr Jessica Eccles seems to be making good progress), one thing is for sure, those with hypermobility seem to have a higher frequency of anxiety disorders and a higher intensity of physiological anxiety in general. Exercise can be a powerful tool when it comes to anxiety, as exercise activates frontal regions of the brain responsible for executive functions, helping control the amygdala, our innate reacting system to real or imagined threats to our survival. As well as benefits to executive functions, exercise also helps to get the heart rate up. This changes brain chemistry, increasing the availability of important anti-anxiety neurochemicals, including serotonin, gamma-aminobutyric acid (GABA), brain-derived neurotrophic factor (BDNF), and endocannabinoids.
Additionally, regular, mindful exercise, can lead to improved joint control, reducing the frequency of dislocations and helping managing pain. I’ve been touted this for a long time, and it’s not just me, esteemed professionals like Rosemary Keer and Dr. Jane Simmonds, alongside the Ehlers Danlos Syndrome Charity UK, are all advocates for exercise for hypermobility. They’re not just preaching about beefing up muscles; they’re talking about a deeper, more mindful way of working with our unique bodies to help understand where they are in space and time.
Muscle Building: An Old Perspective
For decades now, emphasis has always been placed on building muscle to help support lax hypermobile joints through exercise, however, if you stop to think for just a moment, this perspective starts to break down fairly fast. There are no shortages in magazine articles or indeed people, who push the agenda of “just build muscle to help support the joint”. Whilst this is not a bad idea, it does have several flaws.
Firstly, building muscle takes a little while and there is a debate of how much muscle individuals can gain, as it depends on several factors including genetics, training intensity, consistency, diet, age, and gender. For men, the potential muscle gain in the first year of dedicated training can range from 15 to 25 pounds, with the possibility of adding another 10 to 15 pounds in the second year. For women, the expected gains are about half of that for men, with 8 to 12 pounds in the first year and another 4 to 6 pounds in the second year.
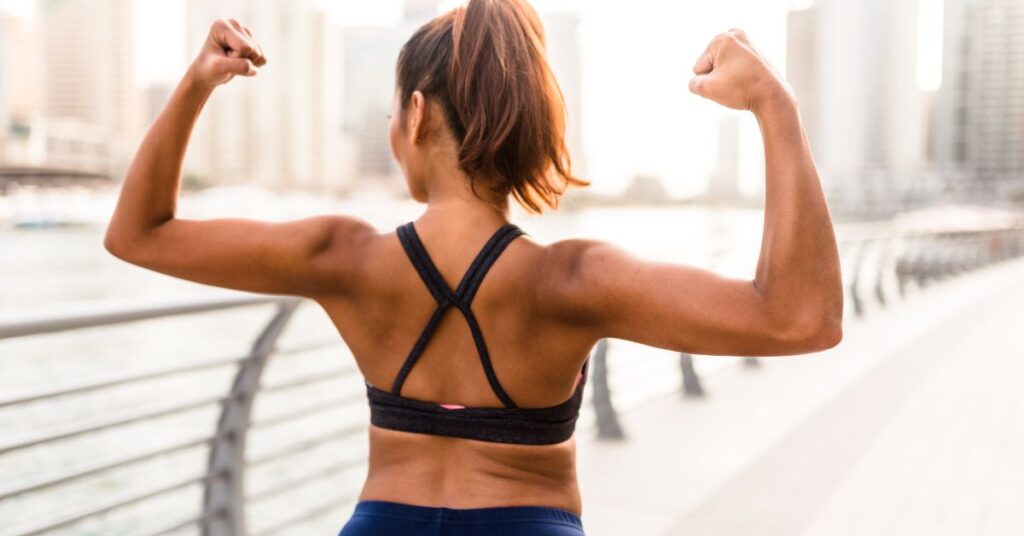
But, again, this will vary wildly person to person, and surprise, surprise, to date there have been no studies that look into this for the hypermobile population, but it is widely medically accepted that muscle strength can help to support joint stability. So then, my first issue here is “How much muscle does someone with hypermobility need to build to stabilise a joint?”
Obviously, there is no answer, because in the grand scheme of things it not particularly important. There are many with hypermobility who have lots of musculature who repeatedly dislocate their joints, and many with much less musculature who don’t dislocate at all. The muscle-to-dislocation ratio just doesn’t correlate.
Likewise, for structures like the Sacroiliac (SI) joint, there are no muscles that directly span it, only several muscles and ligaments provide support and stability to this area. So then, how is building muscle going to help?
Secondly, building muscle has a few prerequisites. You need good nutrition, which should be rich in protein to support muscle repair and growth, as well as balanced with carbohydrates for energy and healthy fats for overall well-being. Adequate hydration is essential to support all bodily functions, including muscle recovery and growth. And consistency in training, with a focus on progressive overload of weight, is also necessary to continually challenge the muscles and stimulate growth.
But it is this last point that causes some issues!
How can one who is suffering from joint subluxations/dislocations, be consistent at lifting heavier and heavier weight when the joints are already unstable and recurrent injuries are ever prevalent?
This contradiction has always been there and is indeed a significant hurdle in managing hypermobility through just muscle strengthening alone. Obviously, some may argue that the weights don’t need to be that heavy, however, muscles only respond to progressive and consistent stress, and if you use weight that is not challenging then it simply won’t lead to muscle building.
For those with hypermobility, who don’t subluxate or dislocate, then muscle building and strengthening exercises are likely going to help, it is just the folks who are somewhat laxer, who may encounter problems with this approach. And with working with the hypermobile population for as long as I have, this is a story I have heard time and time again.
When you stop to think about it, this advice seems a little flawed and doesn’t make much sense when you start to drill into it.
Proprioception: A Slightly Newer Perspective
Proprioception and hypermobility are two words that get clumped together as commonly as “fish and chips” does here in the good old UK. It’s also a term that gets banded about and often used as a buzz word, when In reality it is only a word that describes the effect of various things working together. Very often, this word makes me want to slam my head into a wall, when people use it incorrectly or out of context. I am, after all, a stickler for semantics.
Proprioception is the body’s ability to sense its position, motion, and equilibrium in space, allowing for coordinated movement and balance without the need for direct visual input.
You may hear a lot of people talk about working on proprioception when referring to hypermobility rehab or hypermobility exercises. However, proprioception isn’t something you directly work on because it is the effect of multiple systems working together, I know it’s a nuanced perspective, but I feel it’s important to draw the distinction. It is essentially a baked cake, it’s not about the cake but the ingredients that go into it: the cake is just the product.
Proprioception is fundamentally the cake to the following ingredients: accurate sensory input, well-developed cortical maps, neural processing, motor skills and function, good tissue health and tone, and don’t forget, good old predictive processes. At 11 million sensory inputs per second, brains are awfully good at picking and choosing what to focus on, and essentially just guessing things.
Considering the complexity of proprioception, it’s clear that exercises labelled specifically for proprioception enhancement may not directly target the underlying mechanisms.
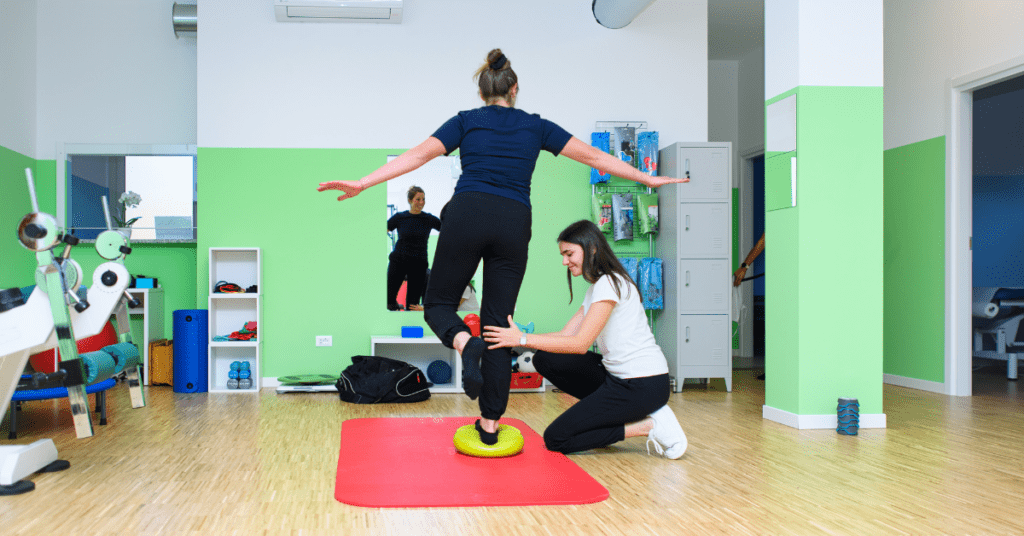
While activities like using a wobbleboard might engage some aspects important for proprioceptive feedback, their real-world applicability is limited. This is akin to improving skills in one musical instrument, hoping it will transfer to mastery in another; while both involve musicality, the skills are not directly transferable.
Thus, this kind of focus when talking about hypermobility and exercise, is at least a better approach than a purely muscle-building one, however, the combination of both would at least strengthen the areas one another lack in, but still not fully address all that needs to be addressed.
Hypermobility and Exercise: How I would love you to view it
So, we mentioned about the ingredients to our proprioception cake, and that to increase proprioception, we need to focus on what goes into the cake, rather than the cake itself. This section then, is more focused on the ingredients, and will hopefully give you a different lens to view things through. This is a topic that can get very confusing, very fast though, so I’m going to try my best and keep it close to the main points, without going into too many unnecessary details, going off on a tangent or branching off too much.
However, this article is going to be big. I haven’t written it yet as I write this sentence, but I already know it’s going to be a monster.
But, to you reading at home, my hypermobile friends, Knowledge is power!
I really do implore you to read through the heavy science parts that come next, because it will let you understand your body better, and it will connect a lot of dots about why traditional training doesnt work all that well when you throw hypermobility into the mix.
So, if you are a little nerdy pants and love absorbing knowledge like myself and the rest fo the team, then strap on in sunshine!
What goes into proprioception?
As I mentioned earlier, the brain receives around 11 million pieces of information per second. The types of sensory input can vary wildly, with different nerves and organs carrying very different information to the central nervous system and brain.
An overview of sensory input
Sensory nerves and receptors can be classified based on the type of stimuli they respond to, which include:
1. Mechanoreceptors: These sensory nerves are sensitive to mechanical stimuli such as pressure, vibration, and touch. They are found in the skin, muscles, and various internal organs. Mechanoreceptors help us recognise textures, maintain balance, and detect changes in muscle, tension or blood pressure. Some examples of mechanoreceptors include Merkel cells (responsible for light touch), Pacinian corpuscles (vibration detection), and Ruffini endings (detecting skin stretch).
2. Nociceptors: These sensory nerves are responsible for detecting potentially harmful stimuli, such as extreme temperatures, excessive pressure, or harmful chemicals, and signalling the CNS to generate a pain response. Nociceptors are found throughout the body, including the skin, muscles, and internal organs. They help protect the body from injury by alerting the brain to potentially damaging situations.
3. Photoreceptors: These sensory nerves are found exclusively in the retina of the eye and are responsible for detecting light stimuli. Photoreceptors convert light energy into electrical signals that the brain can interpret as visual information. There are two main types of photoreceptors: rods (responsible for low-light vision) and cones (responsible for colour vision and high visual acuity).
While the categories above cover the main sensory nerves, there are additional subtypes and specialised sensory nerves, receptors, and cells within these categories that perform specific functions. Some of these include:
1. Proprioceptors: A subset of mechanoreceptors, proprioceptors are found in muscles, tendons, and joints. They provide information about the position and movement of body parts, allowing for proprioception or the sense of body position and movement. Key types of proprioceptors include muscle spindles (detect changes in muscle length) and Golgi tendon organs (monitor muscle tension).
2. Auditory hair cells: Found in the cochlea of the inner ear, these specialized mechanoreceptors are
responsible for detecting sound vibrations and converting them into electrical signals that the brain interprets as auditory information.
3. Free nerve endings: These are unspecialized nerve endings found throughout the body that can respond to multiple types of stimuli, such as pressure, noxious stimulus, and temperature. Free nerve endings are considered polymodal nociceptors since they can detect different types of potentially harmful stimuli.
While this list is not exhaustive, it does cover the major types and subtypes of sensory nerves. As you can probably appreciate now, the nervous system is a complex and diverse network, and there are many specialised sensory nerves and receptors that work together to help us perceive and respond to our environment.
Mechano Receptors GTO and Muscle Spindles
Now that we have a good overview of the sensory information coming in, I would like to dive a little deeper into some of the more important receptors we covered, and a good place to start is with the traditional “proprioceptors”.
Golgi Tendon organ
Let’s start with the Golgi tendon organ, and a quick point to note is that the term “organ” can often be confusing, seeing as it is not an organ in the traditional sense. Its name comes from historical context and the structure’s appearance, rather than reflecting its classification as an organ like the heart or liver.
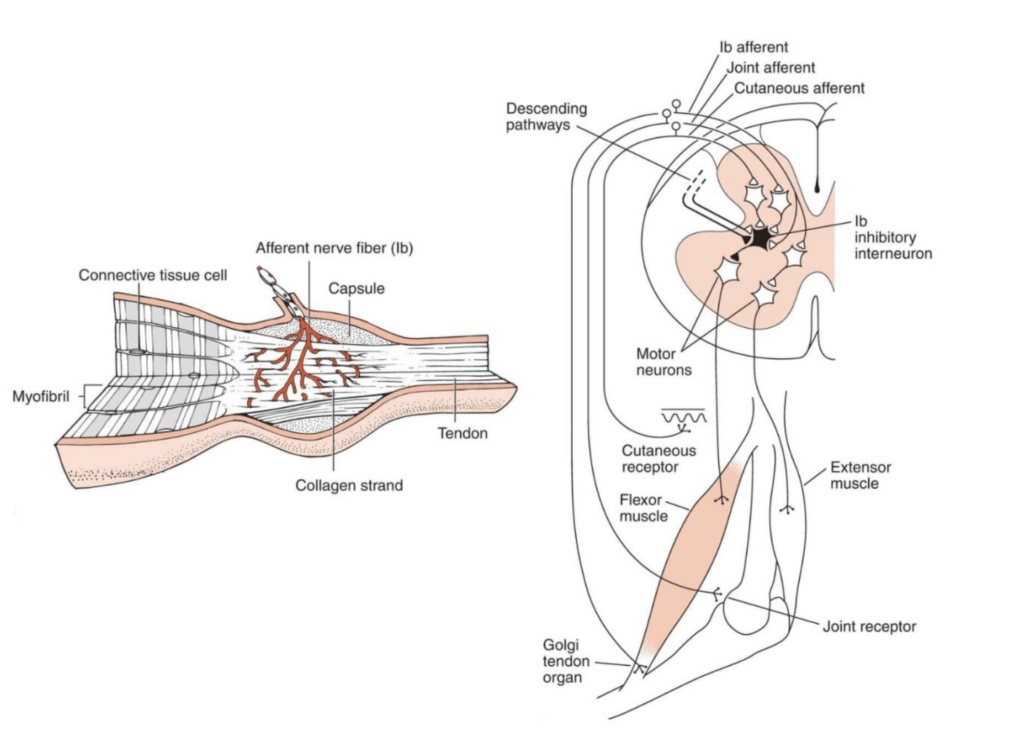
Golgi tendon organs are named after Camillo Golgi, an Italian physician and scientist who made significant contributions to the understanding of the nervous system. The term “organ” in this context refers to thestructural arrangement of nerve fibres and collagen within the tendon, creating a functional sensory receptor unit.
So, even though the name “Golgi tendon organ” contains the word “organ,” it is actually a specialised sensory receptor, not a distinct organ like those found in other body systems.
The GTO is essentially our body’s own quality control system for muscle tension. It’s stationed at the junction where muscle meets tendon, perfectly placed to monitor the tension that could potentially lead to overexertion and injury. Think of it as a diligent sentry, keeping a constant watch over the forces coursing through our tendons.
The magic begins when our muscles contract or stretch. This action pulls on the tendons, which in turn, squishes the GTOs nestled within. Each GTO is made up of collagen fibres and nerve endings (Ib afferent fibres, to be precise). When the collagen fibres are deformed due to muscle tension, it activates these nerve endings. These nerves then shoot off signals to the central nervous system (CNS), carrying real-time updates on muscle tension.
This system allows the CNS to make split-second decisions, like adjusting muscle tone or, in extreme cases, initiating a reflex to prevent muscle damage by reducing the tension (thanks to the inverse myotatic reflex).
For those with hypermobility, the GTO’s feedback is crucial yet complicated. Our ‘laxer’ tendons can mean the GTO’s signals might not be as clear-cut as they should be. It’s akin to receiving a fuzzy radio signal when you’re trying to tune into your favourite station. There is also a few things that can impair how well this sensory information fires:
- Muscle Tension: The GTO is finely tuned to the ebb and flow of muscle tension, sending signals to relax the muscle when it senses too much strain, akin to letting out a breath you didn’t know you were holding.
- Muscle Fatigue: Tired muscles affect the GTO’s performance, altering its communication with the brain and potentially leading to less stability and more injury risk.
- Adapted Movement Patterns: Our bodies are masters of adaptation, finding ways to move that minimize discomfort. These adaptations can influence the GTO’s feedback, affecting how muscles and tendons bear the load.
- Injury or Inflammation: Swelling or injury can skew the GTO’s sensitivity, disrupting its ability to accurately judge muscle tension.
- Aging: With age, changes in muscle and brain function can impact the effectiveness of the GTO’s feedback system.
- Collagen Laxity: In conditions like EDS or JHS, the unique structure of collagen can affect the GTO’s sensitivity to tension, posing challenges for joint stability.
Muscle spindles
To complement out GTO which are monitoring muscle tension, Muscle Spindles keep track of how much a muscle stretches and how quickly it happens. They’re made up of special fibres called intrafusal fibres, snugly situated among the regular muscle fibres (extrafusal fibres) that do the heavy lifting.
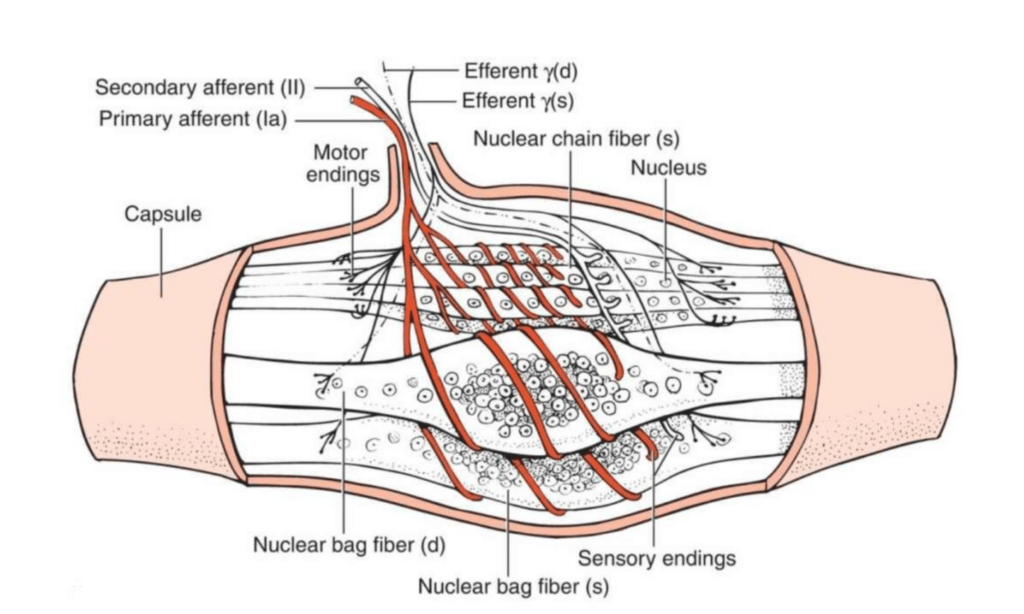
These intrafusal fibres come in two delicious flavours:
- Nuclear bag fibres: Think of these as the dynamic detectors, quick to respond to how fast a muscle changes length.
- Nuclear chain fibres: These are the steady sentinels, more focused on how much the muscle has stretched overall.
Wrapped in a protective sheath, these fibres form the muscle spindle itself. Positioned parallel to the main muscle fibres, they’re perfectly placed to sense any stretching action.
Muscle spindles also have a direct line to the brain, thanks to two other types of nerve fibres:
- Primary (Ia) afferents: The rapid responders, alerting to quick changes in muscle length.
- Secondary (II) afferents: The detailed reporters, focusing on the steady state of muscle stretch.
Moreover, muscle spindles have their own motor supply through gamma (γ) motor neurons, which fine-tune the spindle’s sensitivity, ensuring that our internal measuring tapes are always calibrated just right. This essentially means they give our central nervous system (CNS) up-to-date sensory information on muscle movements, helping with things like:
- Proprioception: Knowing where our limbs are without looking.
- Muscle Tone Regulation: Keeping muscles ready but not overly tight.
- Joint Stability: Especially crucial for those with hypermobility, where ligaments and joints might be a bit too lax.
The Stretch Reflex
Muscle spindles also trigger the stretch reflex, a quickfire response that contracts a stretched muscle and relaxes the opposing one, guarding against overstretching and injury.
For those with hypermobile, muscle spindles are a very important receptor, given our extra-flexible joints, as the feedback from muscle spindles helps compensate for what our ligaments and joint capsules lack. However, as normal, hypermobility can throw a spanner in the works:
- Joint laxity might affect how muscle spindles sense and respond to changes, potentially leading to reduced sensitivity or altered feedback.
- Injury and guarding behaviour can tweak the firing rate of these spindles, impacting muscle tone and reflex responses.
It’s also important to note that Muscle spindles don’t operate in isolation. Changes in one muscle can affect neighbouring ones, thanks to interconnected tissues. This interconnectedness means that a tweak here can lead to adjustments there, highlighting the complexity of maintaining balance and stability, especially in hypermobile bodies.
Cutaneous nerves
The skin, a marvel of human biology, stands out as perhaps our most sensitive organ. It’s a testament to how tactile we are as beings, constantly interacting with the world through touch. The myriad of nerves nestled within our skin layers are ceaseless informants, each relaying different types of sensory data back to the brain, painting an internal picture of our body’s position and movements.
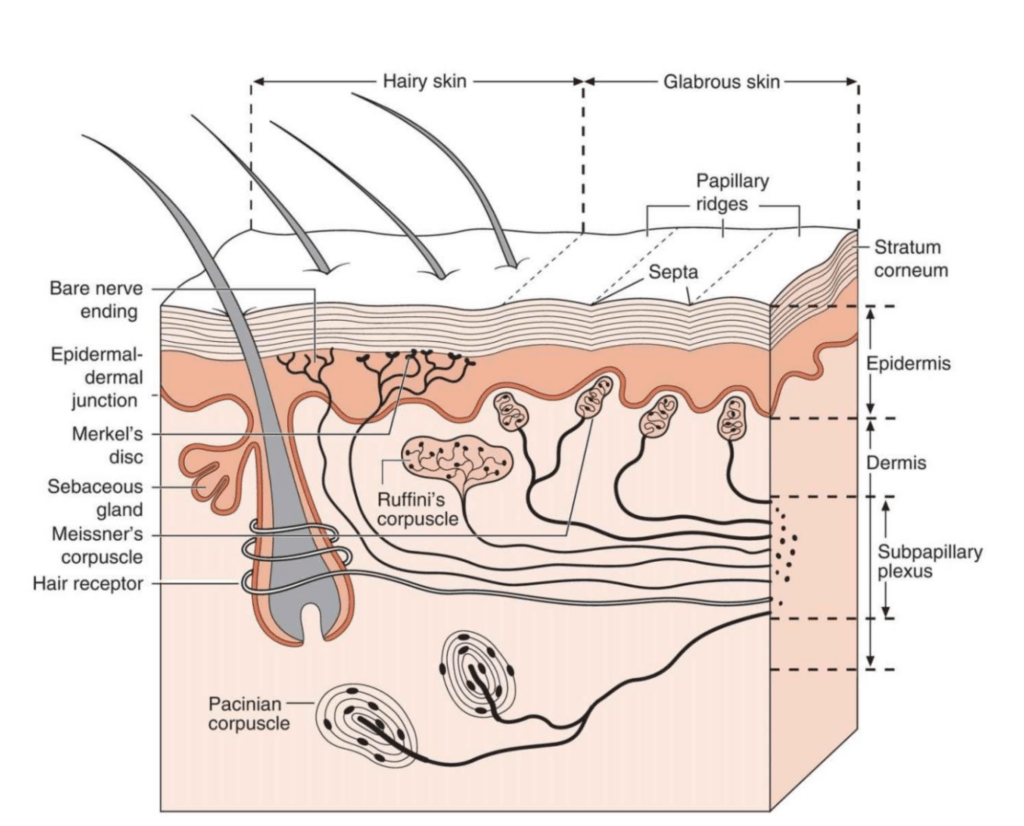
Take a simple action, like flexing your hand. As you do this, the skin on the back of your hand stretches—a sensation keenly noted by specific nerves dedicated to detecting this change. These nerves promptly dispatch this information to the brain. Simultaneously, the skin on the palm of your hand experiences compression, a change that does not go unnoticed. The nerves in this area also send their reports to the brain. This dual feedback of stretching and compression provides a nuanced snapshot of the hand’s movement.
But the story doesn’t end with the skin. This sensory information is combined with inputs from other players in the proprioception game—the Golgi tendon organs (GTOs) and muscle spindles. The GTOs keep an eye on the tension within muscles, while muscle spindles monitor how much and how fast muscles stretch. Together, this ensemble of sensory data converges in the brain, allowing it to decipher with remarkable accuracy where our hand is in space and time.
For a handy little list of our sensory nerves and what they do, see below:
- Meissner’s Corpuscles: Located in the superficial dermis, particularly in areas like the fingertips, palms, and soles of the feet, these receptors are sensitive to light touch and vibrations of low frequency. They help in detecting textures and fine details through touch.
- Pacinian Corpuscles: Found deeper in the dermis and in some subcutaneous tissue, these are sensitive to deep pressure and high-frequency vibration. They help in perceiving the sense of when an object is vibrating against the skin or changes in joint position that apply deep pressure.
- Merkel’s Disks: Located in the superficial layers of the skin, these receptors are sensitive to light pressure, allowing for the detection of shapes, edges, and textures in detail.
- Ruffini Endings: Situated in the deeper layers of the skin and in the ligaments and tendons, Ruffini endings respond to sustained pressure and skin stretch, contributing to the perception of object manipulation and finger position, thus indirectly supporting proprioception.
- Free Nerve Endings: These are the body’s all-rounders, found throughout the epidermis and some parts of the dermis. Free nerve endings are versatile, capable of detecting temperature, dangerous stimulus, and even subtle touches. Unlike the more specialised nerves, they provide a broad range of sensory feedback, making them polymodal.
The Vestibular System
Alongside nerves and sensory receptors like the GTO, the vestibular nerves are responsible for transmitting information about the body’s position, movement, and balance to the brain.
Imagine the vestibular system as one of the key puzzle pieces in understanding our body’s orientation. It’s not just about not falling over; it’s about knowing where every part of us is in the world around us, without having to look. For those of us with hypermobility, this is vital. Our joints may not always give us reliable feedback, so we lean heavily on this internal guidance system, and if there are problems hear (did you see what I did there) then it often causes a lot of problems with proprioception.
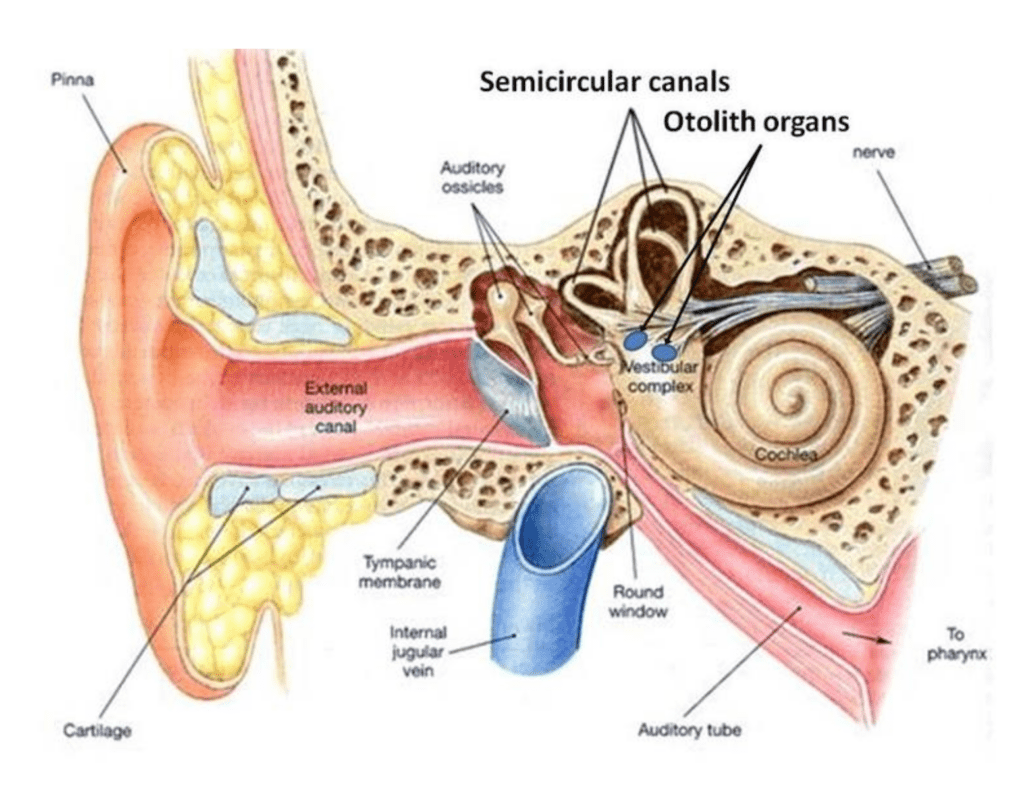
At the heart of this system are the vestibular nerves, branching out to connect with tiny, highly sensitive cells that detect movement and changes in head position. These cells are our direct line to understanding motion, acceleration, and even the direction we’re facing. They work in tandem with the proprioceptive feedback from our muscles and joints, along with visual cues, to create a comprehensive sense of balance and spatial orientation.
The vestibular system’s components, including the utricle, saccule, and semicircular canals, are like the sensors that feed information to our brain’s control centre. They tell us if we’re upright, tilting, accelerating, or spinning. This feedback is crucial, especially in a hypermobile body where the usual cues from joints might be a bit off. It helps us adjust our posture, stabilise our gaze, and move smoothly.
The Visual System
The Visual system gives us a continuous stream of, very reliable and salient sensory input, that informs the brain about the body’s position in its surroundings. This input is critical for everyone, but it plays an especially pivotal role for individuals with hypermobility, where other proprioceptive feedback may be less reliable. For a brain, the eyes never lie.
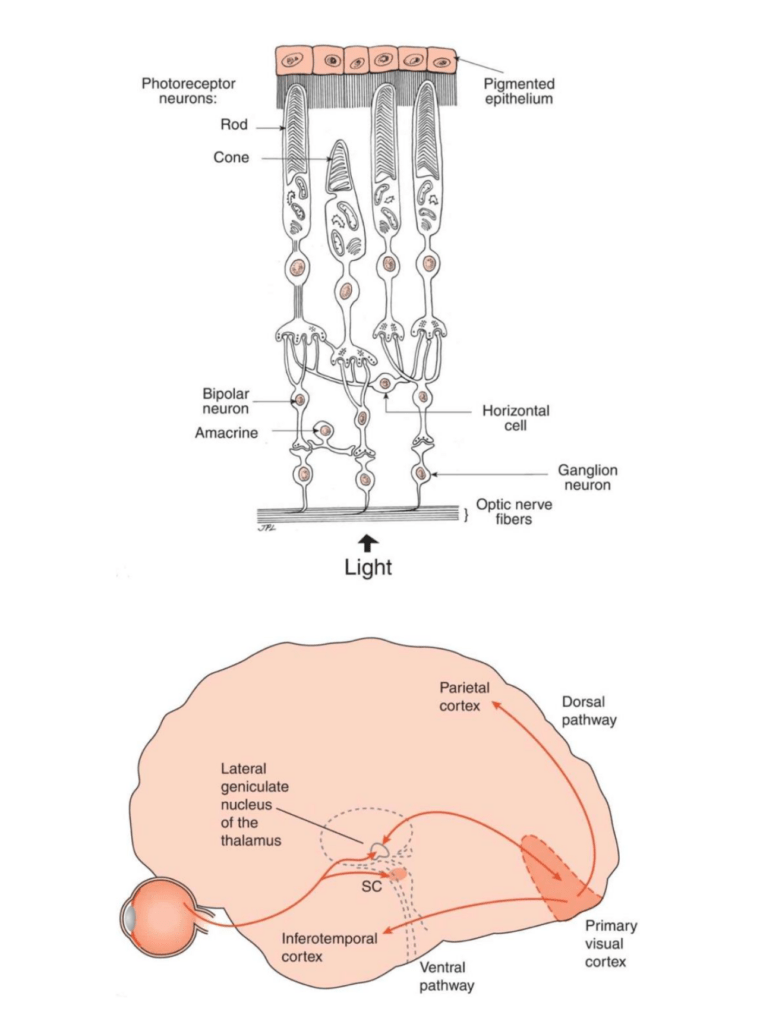
Now, this section could be monstrously long, but it is rather unnecessary, so I would like you to focus on 3 main things:
- Light Reception: It all begins with light hitting the retina, where photoreceptor cells (rods and cones) detect and convert light into electrical signals. Rods excel in low light and provide black and white vision, while cones are responsible for colour vision and detail in well-lit conditions.
- Signal Processing: These electrical signals are then processed by other retinal cells (bipolar cells, horizontal cells, and amacrine cells) that start to interpret shapes, movement, and patterns. The integration of signals from multiple photoreceptors enhances contrast, motion detection, and spatial resolution.
- Transmission to the Brain: The processed signals are relayed to the brain via the optic nerve. Before reaching the visual cortex in the occipital lobe, where visual processing culminates, part of this information is shared with the brain’s balance centres (the vestibular nuclei and cerebellum) and regions responsible for coordinating movement.
The visual system can effectively help compensate for the proprioceptive ambiguity caused by the lax joints found in hypermobility, by providing detailed information about the environment, visual input helps the brain make informed decisions about posture and movement, enhancing stability and reducing the risk of injury. This is one of the reasons why when people with hypermobility often get distracted or have a lapse in concentration, subluxations and accidents can occur more easily.
Cortical Maps
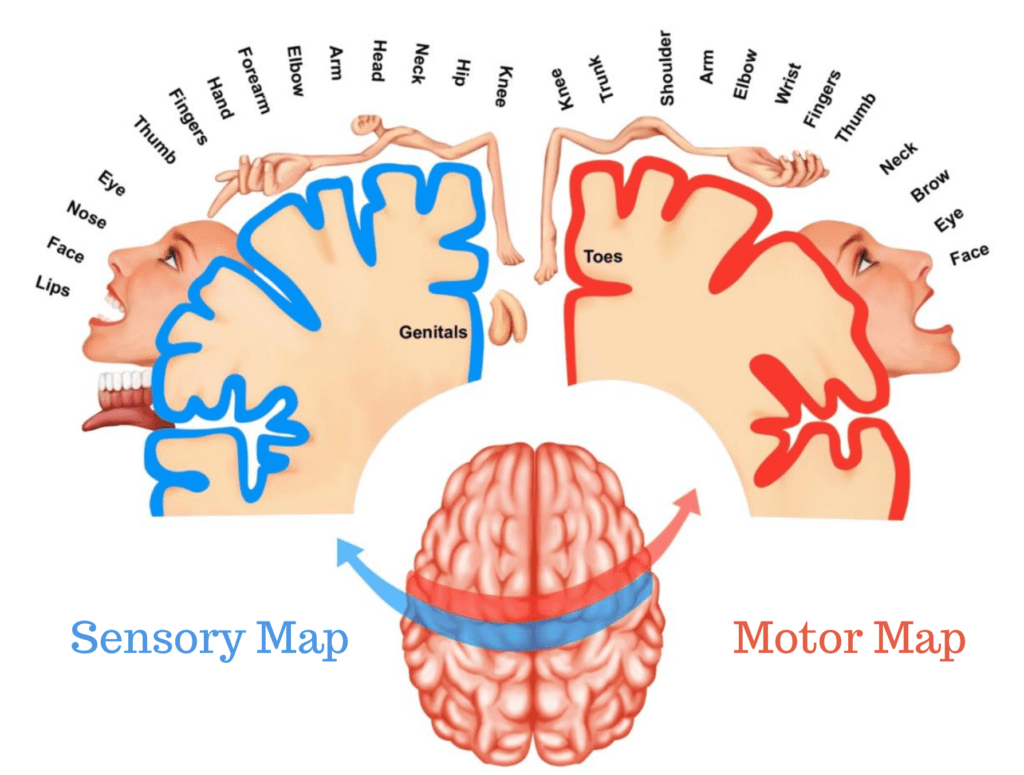
The Somatosensory Cortex (The Sensory Map)
All of that sensory information that we just discussed has to go somewhere, and the place it does go to is your somatosensory cortex, or as we will refer to it from now on: your sensory map. When sensory input zips into the brain, it’s not just dumped into a giant bin labelled “sensations.” No, it’s far more organised and sophisticated than that.
The Sensory Map is located in the postcentral gyrus of the parietal lobe and it’s the main player here. It’s like the sorting centre where sensory input is processed, understood, and then sent off to inform our every move, thought, and reaction.
Think if it like this, when you boot up google maps looking for a pizza place, each Pizza shop on google maps represents a real physical pizza shop in the world around us. Your sensory map is no different, each part of your body that you can feel, is represented within your sensory map. Moreover, this map isn’t static; it’s dynamic, constantly updating based on sensory experiences, injuries, and learning.
We are actually born with the basic framework of these maps, so regardless of how many limbs you were born with, the map you inherent at birth will have two arms and two legs. This is one of the reasons why those with amputation may end up in pain or feeling strange sensations in limbs that are no longer there. Because truth me told, when you feel a sensation, you aren’t feeling it in the part of the body being touched, you are feeling it in the brain, in the area your brain thinks that body part is. However, our sensory map is refined and detailed through experience. It’s a bit like being handed a sketch of a map at birth, with life’s experiences colouring in the details, roads, and pathways. The more we use a sensory or motor pathways, the more detailed that part of the map becomes.
But, not all body representation in the brain are equal, with some body parts being over represent and tasking up more brain real estate than others. It all boils down to use and necessity. More sensitive areas that require finer control, like the fingers for typing or the tongue for speaking, command larger areas on the sensory map. This allocation of cortical real estate allows for more precise control and feedback from these highly utilised body parts.
A Detailed Map Equals Better Proprioception
A well-defined cortical map enhances proprioception by providing the brain with a clearer picture of the body’s position in space (see what I meant about proprioception being the end result?). Think of it as the difference between navigating with a high-resolution map versus a blurry, an undefined one. The sharper the map, the better the brain understands where each part of the body is and how it’s moving, leading to improved coordination, balance, and movement efficiency.
For those of you old enough to remember analogy television, it’s a little like when you would knock the TV ariel and the picture would go static and fuzzy. You would have to wiggle the aerial to help tune the picture in to make it clearer, this is essentially what good rich sensory information does to the brain’s cortical maps of your body.
As I mentioned before, cortical maps are not set in stone; they are highly plastic, meaning they can change and adapt based on sensory experiences and training. This plasticity is a double-edged sword. It allows for the refinement of motor skills and adaptation to new sensory inputs or the loss of a limb (phantom limb sensation is a testament to this). However, it also means that chronic pain or injury can “smudge” these maps, leading to impaired sensory perception and motor control. Going back to our analogy TV analogy, prolonged nociception, injuries, or pain, can cause static to our maps, ultimately making it harder for our brain to read our body, and if it can’t do that or doesn’t understand where things are in space and time, it’s going to have a hard time stabilising joints and moving around without banging into things.
So, in a nutshell, all of that lovely sensory information from nerves and receptors that you get every second, well it helps to detail our maps of our body. However, prolonged nociception, pain, and injuries can cause blurring of these maps.
The Motor Map
Shifting gears from the sensory to the motor side of things, let’s look into the role of the motor cortex now, or what we’ll dub here as the “motor map.” This region of the brain plays the big boss of our movements, orchestrating everything from a shrug to a sprint.
Located in the frontal lobe, specifically in the precentral gyrus (just a stone’s throw away from our sensory map), the motor map is where the rubber meets the road for turning thought into action. If the sensory map is about receiving messages from the body, the motor map is all about sending them out. It’s the dispatch centre for all voluntary muscle movements. Imagine it as the control room for directing your body’s every move, from the delicate art of threading a needle to the powerful thrust of a high jumper.
This map, much like its sensory counterpart, is meticulously organized. Each region corresponds to different body parts, but here’s the thing: it’s not just a simple one-to-one correspondence. The real estate on this map is allocated based on the complexity of the movements required, not the size of the body part. That’s why your hands and face hog a lot of the limelight here: they need to perform intricate ballets of movement that demand precise control.
Like the sensory map, it isn’t just a static blueprint; it’s more like a dynamic GPS system that’s constantly updating. Through plasticity, what we talked about earlier, the motor map can rewire itself based on experience and training. This is just one of the reasons why a pianist’s fingers become so swift and accurate over the keys through time, or how a footballer’s feet become so adept at dribbling? That’s plasticity in action, fine-tuning the motor map for peak performance.
The interplay between the sensory and motor maps is an important one, as for graceful movement, both maps need to be in sync, sharing accurate, up-to-date information. When this communication is crystal clear, movements are smooth, efficient, and, frankly, pretty easy. But, let any “static” disrupt this flow, and suddenly, the simple act of walking or reaching for a cup can become an issue.
Additionally, while the motor map plays the starring role in orchestrating our movements, it doesn’t just work in isolation. Surrounding this star are supporting characters, each contributing their unique skills to ensure every motion is smooth, precise, and appropriate for the situation.
Premotor Cortex and Supplementary Motor Area (SMA)
Just beyond the borders of the primary motor cortex lie the premotor cortex and the SMA. These areas are the brain’s strategists, involved in planning movements before they’re executed. They consider the current scenario and decide not only which movements are necessary but how they should be performed. The premotor cortex focuses on movements directed by external cues, while the SMA handles sequential or simultaneous movements that are internally generated. Together, they’re like the directors behind the camera, setting the scene for the motor map’s action.
Association Cortex
This extensive network serves as the brain’s integration centre, combining sensory feedback with motor commands. It ensures that movements are not only executed but also adjusted based on the environment. Like a skilled editor, it fine-tunes the script, ensuring that the actions meet the demands of the surrounding context.
Basal Ganglia
Deep within the brain, the basal ganglia oversee the modulation of movements. This ensemble of nuclei fine-tunes the intensity and inhibits excessive motion, ensuring fluidity and grace. They’re like the producers, working behind the scenes to ensure the show goes off without a hitch, managing the balance between action and cut.
This intricate network, with the motor map at its core, is a testament to the brain’s complexity and its capacity for nuanced control. The premotor cortex and SMA set the stage for movement, the association cortex adjusts the performance based on real-time feedback, and the basal ganglia refine the output, ensuring that each motion is purposeful and precise.
However, just as in any complex system, challenges can arise. Overuse, injury, and pain can disrupt this harmonious operation, leading to a loss of precision and coordination.
Nociceptors
Now, a very important topic for you to get your head around when it comes to hypermobility and exercises, or stable or lax joints, is the role of nociceptors. Earlier I mentioned that all nociceptors are free nerve endings, but not all free nerve endings are nociceptors, and that we would talk about it later. Well, here we go.
Nociception is a fancy word, but its job is straightforward: to alert us to potential harm. This system is sophisticated, relying on specialized nerve cells, known as nociceptors, that are the body’s scouts. These little warriors are stationed all over — in our skin, muscles, joints, and even some internal organs. Their mission? To detect signals of injury, extreme temperatures (both hot and cold), or chemical dangers, and then send an SOS to the brain.
In our journey to understand pain and our body’s response to it, it’s crucial to debunk a common myth: the existence of “pain receptors.” Our body houses nociceptors specialised high threshold nerves that detect potential harm, extremes as it were, in temperature, pressure, stretch, or chemical signals, but they do not directly transmit pain. Pain, in fact, is a complex creation of the brain, an interpretation of the signals sent by nociceptors. This distinction underscores pain as an output generated by the brain, not a direct input from these sensory neurons. In fact, the term “pain signals” is a rather archaic term, something first used when we were still burning those accused of witchcraft at the stake.
However, not all pain is the product of nociception, and nociception and pain are not mutually exclusive with each other.
The Nociceptive Pathway
Let’s break down how this all plays out, using a walking barefoot in the garden scenario. The moment a thorn pricks your foot, nociceptors spring into action. They convert the physical stimulus (the thorn’s prick) into an electrical signal, which zips through the nervous system to the spinal cord and then to the brain. It’s like the body’s version of instant messaging.
Once the brain gets the memo, it interprets the signal, and you feel pain. But it’s not just about causing you discomfort; pain is a call to action. It prompts you to pull away, look down, and address the injury. Without this system, you might not even realize you’ve been hurt, leaving injuries unchecked and potentially getting worse.
Nociception is rather complex, but the main types of nociceptors playing leading roles in the great play that is nociception are:
- A-delta fibers: The rapid responders. Encased in a thin myelin sheath, these fibers swiftly respond to stimuli, prompting a quick withdrawal from the source of discomfort. Their primary role is in the rapid detection of stimuli that could cause harm, facilitating an immediate protective response.
- C fibers: The chroniclers of discomfort. These unmyelinated fibers take a slower approach, gradually informing the body of persistent or lingering stimuli that might indicate a more prolonged source of potential harm. They account for the lasting awareness of discomfort following the initial detection.
- A-beta fibers: Typically, not associated with nociception, these fibers focus on non-nociceptive stimuli. Yet, under certain conditions, they can also contribute to the overall sensory experience in response to intense stimuli, when we get into the realm of central sensitisation.
Nociceptors engage with a spectrum of stimuli: mechanical, thermal, and chemical, each eliciting a unique response within the sensory system:
Skin Nociceptors:
Situated at the forefront, skin nociceptors act as the initial line of defence, identifying harmful external stimuli. Their activation doesn’t directly communicate pain but signals the need for protective action, potentially leading to localized responses like inflammation to safeguard the area.
Thermal Nociceptors:
Specialised in detecting significant temperature deviations, thermal nociceptors help the body react to extreme heat or cold, protecting tissues from temperature-induced damage. They play a vital role in initiating responses to thermal stimuli that could otherwise harm the body.
Joint Nociceptors:
Residing in the depths of joint tissues, these nociceptors are attuned to the signs of mechanical, thermal, and chemical changes that might threaten joint health. Their role is crucial in the context of hypermobility, where joint stability is often compromised.
Nociception and Hypermobility
For those with hypermobility, the experience of nociception can be markedly different and more complex than in the general population. The hypermobility of joints means that tissues are often placed under unusual stress and strain, which can lead to an increased activation of nociceptors, making them fire when they really need not be. This heightened sensitivity can result from both the mechanical stress of joints moving beyond their normal range, injuries, inflammation, or simply the brain being eager to protect you by reducing the threshold that they fire at.
This increased nociceptive activity can serve as a double-edged sword. On one hand, it’s a protective mechanism, alerting you to potential damage or danger. On the other hand, it can lead to chronic pain conditions, where the pain becomes a constant, debilitating presence, and blurring your cortical maps, rather than just a temporary danger message. For anyone who is hypermobile and is trying to exercise to help themselves, they have to understand the role of nociception and how it affect you.
For instance, sickness and illness, when you come down with the flu, that systemic inflammation is going to send a barrage of nociceptive signals to your brain and it is going to affect your sensory map, making you more prone to injuries and far more sensitive to stimuli that you would normally be. It is the little things like this that is the devil in the details when it comes to people trying to make their joints more stable or partake in regular exercise.
Sensory Issues
Another important thing we should discuss is the fact that hypermobility often brings a lot of sensory challenges that significantly affect movement control and joint stability. These challenges can stem from various sources, such as diminished proprioceptive feedback from lax tissues, structural and functional alterations, damage, and heightened nociceptive responses.
Structural Alterations
Hypermobility can stretch ligaments and joint capsules, leading to increased joint laxity and reduced stability. This laxity allows for greater motion but can decrease proprioceptive feedback due to excessive movement, undermining the ability to sense joint position and movement. This situation can lead to joint instability, heightened injury risk, and motor coordination issues. Additionally, abnormal sensory input from proprioceptors, stretched or compressed beyond their design, can lead to:
- Decreased joint position awareness: Difficulty in interpreting sensory input from proprioceptors can diminish the ability to perceive the affected joint’s position in space.
- Compromised motor control: Essential for coordinating muscle actions and controlling joint movements, impaired proprioception can cause challenges with movement coordination, fine motor skills, and maintaining balance.
- Elevated injury risk: Joint instability and impaired proprioception heighten injury risks by hampering the body’s response to sudden joint position or movement changes.
- Muscle imbalances and compensatory strategies: The body may adopt compensatory strategies to manage joint instability, leading to muscle imbalances, overuse injuries, and further joint issues.
Nerve Compression or Impingement
Connective tissue laxity can elevate nerve compression or impingement risks, reducing structural stability. Nerves may be more prone to compression or impingement, causing sensory impairments such as:
- Numbness and tingling: Nerve compression can disrupt signal transmission, leading to numbness or tingling in the affected nerve area, impacting cortical maps.
- Nociception: Nerve compression or impingement can trigger inflammation, altering map accuracy through nociceptive input.
Functional Changes
Hypermobility’s connective tissue laxity and joint instability can alter the firing rate of proprioceptive structures like Golgi tendon organs (GTOs) and muscle spindles, affecting neuromuscular system functions:
- Golgi Tendon Organs (GTOs): These sensors detect muscle tension changes. Altered tension and force patterns can affect GTOs’ ability to sense muscle tension, contributing to reduced muscle control and movement coordination.
- Muscle Spindles: These receptors monitor muscle length and stretch velocity changes. Hypermobility may expose spindles to a wider range of muscle lengths and velocities, potentially reducing their sensitivity.
Low Muscle Tone
Hypotonia can impair sensory feedback due to functional changes in the neuromuscular system, characterised by reduced passive movement resistance, enhanced joint flexibility, and decreased strength. This can lead to:
- Impaired proprioception: Reduced muscle tone can affect proprioceptive receptors, challenging movement coordination and balance.
- Altered joint sensory input: Increased joint flexibility can alter sensory input, affecting proprioception and body awareness.
- Heightened nerve compression risk: Low muscle tone can reduce structural support, increasing nerve compression or impingement risks, leading to sensory impairments like numbness, tingling, or pain.
Fatigue
Hypermobility-associated fatigue arises from the extra effort needed for joint stability and daily activities, impacting muscle endurance, strength, recovery, sleep quality, energy levels, and exacerbating pain symptoms.
Altered Muscle Activation
Hypermobility can cause muscles around joints to activate in unusual patterns to compensate for passive support lack, leading to muscle imbalances, overuse injuries, reduced movement efficiency, abnormal movement patterns, and impaired neuromuscular control.
As you can see, the fact that our tissues are laxer, and the degree of that laxity, can affect a lot of systems that need to work together.
The Subluxation/Dislocation Cycle
When it comes to things that get in the way of exercising with hypermobility, the subluxation/dislocation cycle is up there. Now, not every person with hypermobility is going to experience a subluxation joint or a dislocation, it really depends on the degree of laxity, but there is a huge cohort of those with hypermobility who do, and more often than not it’s this group that gets pushed, sometime forcefully, into hypermobility exercise.
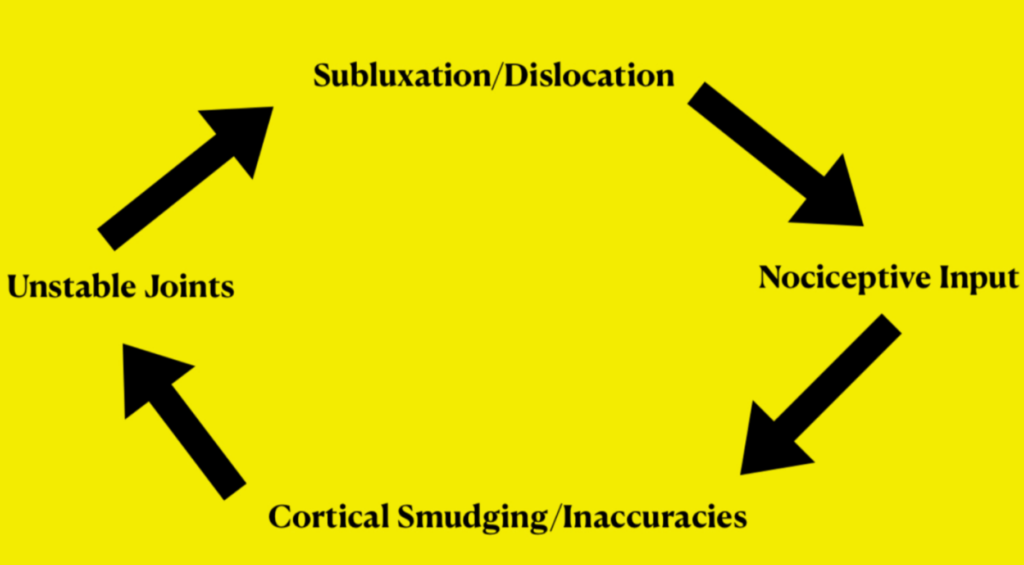
However, it can often be hard to exercise when the following keeps happening in the same order:
A joint Subluxes or dislocates: Either through force or an accident, a joint partially dislocates, or fully dislocates.
The Body’s Alarm System: Nociception: Following a subluxation or dislocation, the resulting overstretching of small ligaments can cause nociception, which alerts the brain to potentially harmful stimuli at the site of the dislocation. The extent of nerve and blood vessel damage, tearing of muscles and ligaments, joint swelling, and nerve compression depends on the severity of the subluxation/dislocation. The important thing to focus on here though, is that nociceptive signals have the potential to disrupt cortical maps as they are given priority over other receptor types, with nociceptors being sensitive to potentially dangerous stimuli, affecting blurring sensory maps and altering motor outputs by ways of limiting motor unit activations to try and keep us safe. Essential, if it’s a bad sublux or dislocation, then the threshold at which nociceptor nerves fire is going to drop, and slight gentle pressure, stretch, etc, is going to cause a nociceptive signal to fire, when it normally wouldn’t. This means we are going to end up with a lot of blurring information going to the brain, and not a lot of map detailing information.
Lack of movement and blurry map: Obviously if you have just dislocated your shoulder or another joint, then in the days after, you are not going to move it a great deal. In fact, you’re probably going to sling it and immobile it, which is the right thing to do: we need that inflammation to do its job. However, an issue that we can’t avoid, is that inflammation is going to make the tissue permeable to help get nutrients in to heal it, but that’s nociceptive, and everything is going to be very sensitive for a while. We then have the fact that we are not going to be moving the joint a great deal because it’s painful, so we don’t really get much in the way of healthy sensory input to help detail our maps to help us stabiles the joint, if anything, we get the opposite: blurrier maps.
Less stable joints: Often enough though, a subluxation or dislocation can be a very scary experience and it can make us fear movement, which is something that can promote guarding postures, change our motor outputs, and even contribute to the development of chronic pain. When you combine that with a map that’s a lot harder for your brain to read to help stabilise a joint, then the chances of that joint subluxation or dislocating increase.
A Quick Recap
I think right here is a good place to hold off on the science and educational parts and a little overview is in order:
Sensory Input and Proprioception in Hypermobility
- Sensory Nerves and Proprioception: We discussed the brain’s processing of an immense volume of sensory information, emphasizing the significance of mechanoreceptors, nociceptors, photoreceptors, and proprioceptors. These sensory inputs are foundational to proprioception, the body’s ability to perceive its position and movement in space. For those with hypermobility, the accuracy and efficiency of these sensory inputs are crucial for managing joint stability and movement.
- Mechanoreceptors: Including Merkel cells, Pacinian corpuscles, and Ruffini endings, these receptors provide critical feedback on touch, pressure, and skin stretch. In hypermobility, the heightened mobility of joints may alter the typical mechanical stimuli these receptors respond to, potentially impacting proprioceptive accuracy and joint awareness.
Key Proprioceptors: GTOs and Muscle Spindles
- Golgi Tendon Organs (GTOs): Serve as monitors for muscle tension, playing a pivotal role in preventing overexertion and injury. In the context of hypermobility, the feedback mechanism from GTOs may be compromised due to the altered tension patterns in lax tendons, affecting the body’s ability to regulate muscle force and protect joints from overloading.
- Muscle Spindles: Track muscle stretch and tension, contributing to the stretch reflex and proprioception. For individuals with hypermobility, the functionality of muscle spindles is particularly important. The altered biomechanics and increased joint range of motion can affect spindle sensitivity and feedback, posing challenges for maintaining joint stability and coordinating movements.
Challenges Stemming from Structural Alterations
Hypermobility can stretch ligaments and joint capsules, leading to increased joint laxity and a reduction in proprioceptive feedback. This laxity undermines the ability to accurately sense joint position and movement, which can escalate to joint instability, a heightened risk of injuries, and coordination difficulties. The abnormal sensory input from overextended proprioceptors complicates the body’s internal mapping of its limbs in space, directly affecting movement control and stability.
Nociception’s Role in Hypermobility
Nociceptors, which signal potential harm to the body, can be overly activated in hypermobility due to the mechanical stress and inflammation associated with joint instability. This increased nociceptive activity not only heightens pain perception but also can blur the sensory and motor cortical maps in the brain, complicating the accurate perception of joint position and movement. This scenario underscores the complex interplay between pain, proprioception, and motor control in hypermobility.
The Subluxation/Dislocation Cycle
This cycle highlights a critical issue in hypermobility: joint instability leading to subluxations or dislocations, which then trigger nociceptive responses and potentially alter neural representations of joint position and movement. The cycle illustrates how joint instability, combined with altered sensory feedback, can exacerbate the challenges of maintaining accurate proprioception and effective motor control, further complicating exercise and movement strategies for those with hypermobility.
Cortical Maps: The Sensory and Motor Perspectives
- The Somatosensory Cortex: Acts as the brain’s sensory map, processing input from all the aforementioned receptors to create a detailed representation of the body’s position and movement. In hypermobility, maintaining detailed and dynamic sensory maps is essential for compensating for the laxity in joints, ensuring accurate proprioception.
- The Motor Cortex: Coordinates all voluntary movements, with its organization reflecting the complexity of required movements rather than the size of body parts. For those with hypermobility, the motor map’s role becomes crucial in executing movements that are adapted to protect unstable joints, requiring precise control and coordination.
However, there is so much more to add to this, as we didn’t even get into how hypermobility affects motor learning or even the brain’s predictive processes, but I feel that perhaps that is another blog’s worth of material. For now, I think the above gives you a good base of education to understand your hypermobile body a lot better, especially when it comes to exercising.
I appreciate that this is a pretty big and heavy article, so I am going to give you some time to digest it. We have gone through what makes up proprioception, and the various factors that are going to cause roadblocks to exercising and stabilising joints when we are hypermobility.
So, for now, take it all in and I will be back very soon for Part 2: practical application!
Stay strong out there!
–The Fibro Guy Team–
Enjoyed Our Blog? Why Stop Here?
If you’ve found value in our posts, imagine what you’ll gain from a structured, science-backed course designed just for you. Hypermobility 101 is your ultimate starting point for building strength, stability, and confidence in your body.